INTRODUCTIONThe vestibular evoked myogenic potential (VEMP) can be generated through auditory, vibratory, or galvanic stimulus.
1 VEMP with auditory stimulation and response capture in the sternocleidomastoid muscle is useful to detect changes in the vestibular system located in the saccule, inferior vestibular nerve, and medial vestibular spinal tract.
1 However, this test does not differentiate peripheral vestibular disorders from central disorders.
The VEMP obtained by galvanic stimulation has the advantage of acting on the postsynaptic membrane along the vestibular nuclei; when combined with other vestibular tests, it allows for differentiation between peripheral or central vestibular alteration.
1-3 The galvanic vestibular stimulation (GVS) of the mastoid process acts directly on primary afferent discharges from the distal portion of the vestibular nerve and vestibular nuclei. In a binaural and bipolar configuration, when applying electrical stimulation to both mastoid processes, the vestibular afferents from the negative side (cathode) are excited, and those from the positive side (anode) are inhibited, by changing the resting potential.
1,2 The stimulus reaches the descending vestibular-spinal and reticulo-spinal medullary tracts, generating an electromyographic (EMG) response related to posture that can be captured through surface electrodes.
1-3 This is the VEMP secondary to a galvanic stimulation (g-VEMP).
GVS interferes with postural response.
3 Changes in the resting potential of the vestibular nuclei cause reciprocal changes in the activity of trunk and lower limb muscles on both sides, resulting in bodily deviation toward the anode, followed by a correction movement. These muscle responses to GVS are interpreted as a protective reflex that aims to maintain postural control after an unexpected vestibular stimulus.
2 GVS is able to generate EMG responses only in those muscles engaged in maintaining balance, and there are literature records of EMG responses captured from the sternocleidomastoid, paraspinal, triceps, tibialis anterior, and soleus muscles.
3-6 Depending on the muscle where the VEMP was captured, there is an assessment of the vestibule-spinal response related to the cervical spine (sternocleidomastoid muscle) or thoracic-lumbar (soleus muscle). Thus, this test allows for an assessment of proprioceptive function, and has been used to define the level of injury in spinal cord trauma.
6 The g-VEMP recorded in the soleus muscle produces a biphasic response, characterized by a short latency (SL) component starting approximately 60 ms after the onset of the stimulus, followed by a component of medium latency (ML), of opposite polarity, arising at around 100 ms.
2,7,8 Although recorded consecutively, the two components of the response are generated and conducted by different pathways until they reach the soleus muscle motor neurons.
4 The SL response appears to be triggered by otolithic afferences and conducted by the reticulo-spinal tract, while the ML response is generated by the semicircular canals and central connections associated with proprioception, and it is transported via the lateral vestibulo-spinal tract to the target motor neurons.
4,9 The SL component is more stable with regard to proprioception variations and age range, while the ML component varies according to the intensity of proprioceptive stimulation and its amplitude increases with age.
9-11 It is believed that the ML component is polysynaptic and reflects a response to postural adjustment.
4 To the authors' knowledge, there are no studies in the Brazilian population to define the reference values for g-VEMP with response capture in the soleus muscle.
This study aimed to describe the results of g-VEMP in normal subjects in their sixth decade of life, in order to establish reference values for this examination employing national equipment, comparing the results with the established international values. The choice of age range was due to the fact that this is the range in which diseases related to postural instability most frequently occur, and therefore, this age would benefit more from GVS as a tool for vestibular diagnosis or rehabilitation.
SAMPLE AND METHOD
ParticipantsA total of 13 subjects were selected, three men and ten women, with absence of tinnitus, dizziness, hearing loss, or postural instability, who were submitted to g-VEMP. The age of participants ranged from 50 to 60 years, with a mean of 56 ± 5 (mean ± SD) years. The inclusion of participants was performed by random invitation of individuals who were within the age range of the study, attended to at the outpatient clinic of otolaryngology, with no neurotology complaints when interviewed. Patients with peripheral neuropathy, gait disorders, decreased visual acuity, neurodegenerative and musculoskeletal diseases, previous history of dizziness, or who were using vestibular function-suppressing medications were excluded.
This study was performed in accordance with Resolution 196/96 of the Brazilian National Health Council, and was approved by the ethics committee of the institution where the study was performed, according to the Ethics in Research Committee Edict No. 266/05. All individuals who participated were properly informed about the adopted procedures, signed an informed consent, and were guaranteed their freedom of research participation.
Procedures and galvanic stimulationAll 13 subjects were submitted to g-VEMP. For this purpose, GVS was applied, which was characterized as a direct, monophasic, and rectangular current at an intensity of 2 mA and 400 ms duration (model EvP4/ActPlus,
® Contronics - Brazil). The stimuli were offered at randomized intervals of 4 to 5 s and responses to 120 stimulations were measured. The bipolar current was applied to the mastoid processes through surface self-adhesive electrodes, with diameter of 3 inches (Model CF3200
®, Valutrode = USA).
For the binaural transmastoid stimulation, both current polarity configurations were used: left cathode, right anode (LCRA) and right cathode, left anode (RCLA). The polarity of the stimulus was automatically controlled by a computer and randomized between trials. GVS was applied in four trials of 30 stimuli each, distributed as follows: 30 responses recorded from the left lower limb (15 LCRA stimuli, 15 RCLA stimuli) and 30 from the right lower limb (15 LCRA stimuli, 15 RCLA stimuli). Then the procedure was repeated for all participants to ensure reproducibility, totaling 60 stimuli in each lower limb.
2 During the examination, subjects remained standing on a flat surface, keeping their eyes closed and bare feet together, with the body leaning slightly forward, promoting contraction of the soleus muscle. The subjects were instructed to rotate the head about 90º in the sagittal plane, contralateral to the lower limb from which the EMG signals were collected (Fig. 1), as the responses are stronger in the lower limb contralateral to the head rotation direction.
2 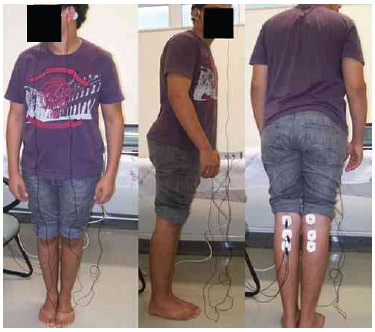
Figure 1 Electrode positioning and subject's posture during examination.
Recording and analysis of electromyographic (EMG) responsesThe EMG activity was measured by means of self-adhesive surface electrodes (Meditrace 300,
® Kendall = USA). Each pair of electrodes was attached vertically, 2 cm below the popliteal fossa, with an approximate distance of 1 cm between them. The reference electrode was placed on the back of the thigh, approximately 3 inches above the recording electrode (Fig. 1). The electrodes were removed from the lower limbs after the end of two trials (30 stimuli for the examination and 30 stimuli for replication), and placed on the other lower limb. A period of rest between trials was allowed to prevent the possibility of muscle fatigue.
The EMG signals were measured, rectified, filtered between 10 Hz and 1 kHz, and digitized at a sampling frequency of 5 kHz. Data were collected over a period of 500 ms, starting 100 ms before the galvanic stimulus. The responses were observed online during the examination (Fig. 2).
2,4 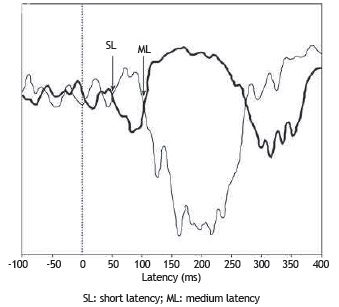
Figure 2 Signals recorded in the right soleus muscle. The thin trace indicates the response from the anode positioned on the right mastoid, and the thick line the response from the anode positioned on the left mastoid.
The analysis of the EMG responses was based on the lower limb contralateral to head rotation. The tracings obtained from the two configurations of electrode placement were superimposed after digital filtering and subtraction of the pre-stimulus EMG rectified mean. The responses that reversed polarity after stimulation for both polarity conditions (LCRA and RCLA) were considered to be of vestibular origin.
2-4 The two components (SL and ML) of the vestibular evoked reflex were defined. A response starting between 40 and 70 ms, which was reversed by inverting the polarity of the stimulus, was considered as the SL component; the ML component had opposite polarity to the SL component, beginning at approximately 100 ms after the stimulus (Fig. 2).
With the overlap of tracings with reverse polarity, the definition of onset of SL and ML components was based at the point where the lines diverged from the basal line of the electromyographic tracing, visually identified and measured by the cursor line. The first divergence between the signals was marked as the beginning of the SL response. Shortly thereafter, the signals returned to baseline and diverged again. The second divergence between them marked the beginning of the ML component. The end of this response was defined as the point at which the signals returned to baseline (Fig. 2). The mean number of the two responses replicated for each participant was calculated to obtain a single value for SL and ML components.
Data analysisThe pattern of response of the two replicable components and the wave latency were the parameters considered for the analysis of g-VEMP. The amplitude was not considered as it is a parameter that varies with muscle tone, thus showing great variation in subjects at the age range of 60 years. Statistical analysis was performed using the Statistical Package for Social Sciences (SPSS - Chicago, US.), release 18.0 for Windows. Measures of central tendency and variability were used to describe continuous variables, and Student's t-test was performed to compare the variables, with a significance level of 5% (p = 0.05).
RESULTSThe g-VEMP was obtained in both legs of all tested subjects, with a total sample of 26 legs. Regarding the shape of the waves, the g-VEMP evoked responses with the same pattern, including SL and ML components and wave inversion by reversing the polarity in all of the 26 evaluated legs. An example of response pattern obtained is shown in Figure 2.
The component SL appeared at a mean latency of 54 ms ± 5, with a minimum of 49 ms and a maximum of 62 ms. The ML component started at a mean latency of 112 ± 8 ms, with a minimum of 98 ms and a maximum of 124 ms.
The variables related to the g-VEMP responses were compared as to side of response recording (Table 1). No difference was observed when comparing the latencies regarding laterality (p > 0.05).
DISCUSSIONThe g-VEMP allows for obtaining the proprioceptive response of muscles engaged in maintaining body balance, including the lower limb muscles.
1 Thus, this test allows for the assessment of postural response related to the vestibulo-spinal tract. The g-VEMP has clinical applicability for the diagnosis of vestibular-spinal pathway alteration and to improve the treatment of patients undergoing vestibular rehabilitation.
2,6,7,9,12 The pattern of the electromyographic waves observed in the present study (Fig. 2) for the soleus muscle was consistent with that observed in previous studies, showing a biphasic response characterized by two waves of opposite polarity, which are known as SL and ML components of the soleus VEMP.
4,13As suggested by Briton (1993), to better identify the responses, the overlapping of the tracings obtained by both stimulus configurations (LCRA and RCLA) is recommended, yielding responses with opposing polarities.
2 This technique, known as "tracing overlap", allowed for the identification of the exact onset of the divergence between the two tracings, facilitating the identification of both response components (SL and ML).
Considering the 26 lower limbs assessed in this study, the first divergence between the signals, defined as the SL component, occurred approximately 54 ms after stimulus onset. The ML response, considered the second divergence, was observed at approximately 112 ms. The response latency found in this study is consistent with previous descriptions (Fig. 2), confirming the vestibular nature of the EMG responses collected, and especially the integrity of the pathway tested by g-VEMP in the assessed subjects.
4,13 Regarding the pathway tested by g-VEMP, until recently, only the vestibulo-spinal nature of responses was considered. Recent studies have brought to light the involvement of the reticulo-spinal tract and, more recently, the difference of conductive stimulus sites.
14 Cathers et al. (2005) observed that the SL component of the reflex response to GVS was triggered by the otolith organs, and the ML component was triggered by the semicircular canals.
10 These researchers have also proposed that the responses were conducted by different projections in the spinal cord, until they reached their target motor neurons; the SL component was evoked via reticular spinal tract, and the ML component via spinal vestibule, which has been reinforced by other studies.
4,13,14 This hypothesis is reinforced by the vestibular system physiology, as there are several connections between the spinal reticular tracts and vestibular nuclei. Thus, the results of the present study demonstrated the functional integrity of the vestibular system in all subjects assessed, including primary afferents of the semicircular canals, saccule, and utricle, in addition to vestibular nuclei, vestibular-spinal tracts, and spinal reticulum.
Changes in the morphology and latency of responses to GVS have been previously observed in subjects with spinal cord injuries.
15 The total absence of both responses occurred in spinal cord trauma.
6 Another situation in which changes are expected in the SL response is in elderly individuals, due to the aging process that causes reduction in a great number of the fast-conduction myelinated fibers.
11,16 Regarding the comparison of the SL and ML component latencies of the response between the lower limbs, no significant differences were observed (Table 1). The responses evoked by vestibular stimulation can actually manifest as slightly asymmetric between the lower limbs, which can be explained by the center of mass shift caused by an unexpected vestibular stimulus. It is known that GVS causes body deviations toward the anode, followed by a correction movement altering the center of mass balance.
17 Regarding response amplitude, these were not analyzed in this study. According to some authors, amplitude is influenced by muscle strength, and may change with age and degree of body inclination,
11 thus it is not a reliable parameter for clinical diagnosis of the vestibular system function. Moreover, studies indicate a change in amplitude with the change in proprioceptive stimuli.
7,9 Muise et al. (2012) demonstrated that the amplitude of the ML component increased after anesthesia induced by cooling of the subjects' feet without neuropathy, with subsequent normalization after the anesthesia effect had subsided.
9 In another experiment, the amplitude of the ML component was reduced when visual information or a tactile support surface was made available.
7 For any of the situations related to the change of proprioceptive stimuli, the SL component remained unchanged.
7,9 These data support the theory regarding the different neural nature of the two components of the soleus response, and indicate the possibility that the ML component can be used as a marker of adequate central integration of sensory responses responsible for body balance.
Considering the robust physiological action of GVS, established clinical applicability and future prospects have been proposed. Promising results have been reported on the use of g-VEMP to differentiate central vestibular lesions,
1 for the functional evaluation of the spinal cord,
6,15 and, more recently, as an auxiliary tool in the treatment of postural instability.
12 GVS can contribute to vestibular rehabilitation due to its effect on vestibular compensation, based on the excitatory effect of galvanic stimulation on vestibular nuclei. Finally, further studies are needed to clarify all the possibilities of GVS action in the diagnosis and treatment of postural instability.
CONCLUSIONIn this study, subjects in the sixth decade with normal vestibular system who received mastoid galvanic stimulation showed a replicable electromyographic response recorded in the soleus muscle that was biphasic, with the first wave at around 54 ms (short latency component), followed by a second wave of opposite polarity at around 112 ms, (medium latency component) of the evoked response. The g-VEMP allowed for the testing of the vestibule-spinal pathway, and is a promising technique to objectively assess the central vestibular system.
CONFLICTS OF INTERESTThe authors declare no conflicts of interest.
REFERENCES1. Watson SRD, Colebatch JG. Vestibular-evoked electromyographic responses in soleus: a comparison between click and galvanic stimulation. Exp Brain Res. 1998:119:504-10.
2. Britton TC, Day BL, Brown P, Rothwell JC, Thompson PD, Marsden CD. Postural electromyographic responses in the arm and leg following galvanic vestibular stimulation in man. Exp Brain Res. 1993;94:143-51.
3. Day BL, Cauquil AS, Bartolomei L, Pastor MA, Lyon IN. Human body-segment tilts induced by galvanic stimulation: a vestibularly driven balance protection mechanism. J Physiol. 1997;500:661-72.
4. Fitzpatrick RC, Day BL. Probing the human vestibular system with galvanic stimulation. J Appl Physiol. 2004;96:2301-16.
5. Baldissera F, Cavallari P, Tassone G. Effects of transmastoid electrical stimulation on the triceps brachii EMG in man. Neuroreport. 1990;1:191-3.
6. Iles JF, Ali AS, Savic G. Vestibular-evoked muscle responses in patients with spinal cord injury. Brain. 2004;127:1584-92.
7. Fitzpatrick R, Burke D, Gandevia SC. Task-dependent reflex responses and movement illusions evoked by galvanic vestibular stimulation in standing humans. J Physiol. 1994:15:363-72.
8. Watson SR, Colebatch JG. EMG responses in the soleus muscles evoked by unipolar galvanic vestibular stimulation. Electroencephalogr Clin Neurophysiol. 1997;105:476-83.
9. Muise SB, Lam CK. Reduced input foot sole skin through cooling differentially modulates the short latency and medium latency vestibular reflex responses to galvanic vestibular stimulation. Exp Brain Res. 2012;218:63-71.
10. Cathers I, Day BL, Fitzpatrick RC. Otolith and canal reflexes in human standing. J Physiol. 2005;563:229-34.
11. Welgampola MS, Colebatch JG. Selective effects of ageing on vestibular dependent lower limb responses following galvanic stimulation. Clin Neurophysiol. 2002;113:528-34.
12. Carmona S, Ferrero A, Pianetti G, Escolá N, Arteaga MV, Frankel L. Galvanic vestibular stimulation improves the results of vestibular rehabilitation. Ann N Y Acad Sci. 2011;1233:1-7.
13. Muto N, Shinomiya K, Komori H, Mochida K, Furuya K. Spinal cord monitoring of the ventral funiculus function. Analysis of spinal field potentials after galvanic vestibular stimulation. Spine. 1995;20:2429-34.
14. Peterson BW, Abzug G. Properties of projections from vestibular nuclei to medial reticular formation in the cat. J Neurophysiol. 1975;38:1421-35.
15. Liechti M, Müller R, Lam T, Curt A. Vestibulospinal responses in motor incomplete spinal cord injury. Clin Neurophysiol. 2008;119:2804-12.
16. Bergström B. Morphology of the vestibular nerve: the number of myelinated vestibular nerve fibers at various ages. Acta Otolaryngol. 1973;76:173-9.
17. Goldberg JM, Smith CE, Fernandez C. Relation between discharge regularity and responses to externally applied galvanic currents in vestibular nerve afferents of the squirrel monkey. J Neurophysiol. 1984;51:1236-56.
1. Faculdade de Medicina da Universidade Federal de Minas Gerais (UFMG), Belo Horizonte, MG, Brazil
2. Universidade Católica de Pelotas, Pelotas, RS, Brazil
Corresponding author.
D.U. Gonçalves
E-mail: d
eniseg@medicina.ufmg.br Received 29 May 2013.
Accepted 30 August 2013.