IntroductionAccording to Ferraro and Durrant (1994), the first recordings of electrical potentials on the human skull as a response to auditory stimuli were made by Davis et al. in 1939. The potentials were generated in the cortex with latencies that ranged from 50 to 500ms. Some years later, thanks to computers, faster and shorter amplitude responses were recorded, known as middle latency potentials, between 10 and 80 ms. These potentials, which precede cortical ones, are supposedly originated in the thalamus and the protection areas of the primary cortex. Brainstem auditory evoked potentials (BERA) were initially identified by Sohmer and Feinmesser in 1967 and later described by Jewett et al. in 1970 and Jewett and Williston in 1971.
Despite its recent discovery and owing to its small amplitude comparing to normal electroencephalic activity and evoked auditory potentials, vast literature has been accumulated about neurological and audiological clinical applications of BERA, especially emphasized on neonatal hearing screening programs (Hood, 1998).
Currently, BERA is a reliable and objective diagnostic tool that has been widely used to early detect hearing disorders. However, despite the significant importance of BERA recordings, obtained by clicks or bursts (transient), especially important to topographic aspects of the hearing disorder, we should bear in mind that the technique has limitations in characterizing residual hearing, which results from the type of acoustic stimulus used - the click, which contains acoustic energy in all frequencies, but is not specific in its spectral content. A second problem is related to cochlear activation pattern by the click, which is circumscribed to a specific region of frequencies around 3,000Hz (Van Der Drift, 1987; Conijin, 1992).
The need to have frequency selectivity in hearing assessment with BERA has motivated different researchers (Gorga, 1988; Stapells-Picton, 1990; Stapells; Gravel; Martin, 1995) to use resources such as: 1) the use of masking associated to clicks to isolated areas of the cochlea and have frequency-specific responses; 2) the use of tone bursts, of specific frequencies, normally named filtered clicks, tone pips or brief tone bursts. However, the difficulty in standardizing stimuli and detection of responses, together with the long time it takes to determine electrophysiological thresholds for each individual frequency, resulted in techniques that are rarely applied in clinical settings (Conijin, 1993).
Even though no reports of clinical application of steady-state evoked potentials (SSEP) have been found in Brazil, the technique initially described by Aoyagi et al. in 1993, has been widely and successfully used in Canada and Cuba (Valdes; Perez-Abalo; Martin, 1997) to objectively detect audiometric responses, especially in high risk children screening and in cases in which conventional tests do not present satisfactory results.
What has attracted the researchers to steady-state potentials (Aoyagi; Kiren; Kim, 1993; Richards; Tan; Cohen, 1994; Lins; Picton; Champagne, 1995, Valdes; Perez-Abalo; Martin, 1997) is the possibility to have a comprehensive audiometric profile by testing each specific frequency without significantly increasing the duration of the test. These tests enable a more detailed and objective assessment of hearing and allow the configuration of an electrophysiological audiogram. Easy recording and objectivity in identifying responses by using standardized statistical analysis, are only some of the important aspects of this technique (Lins; Picton; Boucher, 1996).
Auditory steady-state evoked potentials are electrophysiological responses to amplitude-modulated tones that can be recorded by surface electrodes, such as the other cortical or brainstem evoked potentials.
According to Lins et al. (1995), steady-state potentials for amplitude-modulated tones of 80-110Hz can be easily recorded in the human skull, present a clear relation with different acoustic variables, provide important data to physiological assessment of the nervous system and can be very useful in objective audiometry because they are obtained in intensities close to those of the thresholds. These responses produced by frequency-specific stimuli are more appropriate to be audiometrically explored that those generated by clicks, favoring better identification of hearing loss audiometric profile.
Sound causes polarization and depolarization of inner hair cells, but only depolarization produces action potentials in the auditory nervous fibers, so the cochlear output contains a rectified pattern of acoustic stimulus. The rectification results in a response generated by a continuous sound that is amplitude-modulated, but can be detected as a spectral peak in the modulation frequency (Aoyagi; Kiren; Furuse, 1994).
A complex acoustic signal, formed up of multiple tones or carrier frequencies, for example, 500, 1000, 2000 and 4000Hz, modulated in amplitude, in which each tone has a specific modulation frequency, can stimulate the different regions of the basilar membrane, that is, it activates a group of hair cells for each carrier frequency.
Modulated tones are independently rectified in the inner ear generating differentiated spectral components (Figure 1) which are represented by a series of peaks, corresponding to the modulation frequencies. The components, which are not part of the spectral content of the stimuli, are used to access the cochlear response to each stimulated frequency. Thus, through the spectral recording of electrophysiological events evoked by amplitude-modulated tones and the use of mathematical procedures to analyze responses in the frequency domain, it is possible to clearly and objectively identify SSEP.
John et al. (1998) examined spectral components of steady-state based on amplitude-modulated stimuli and concluded that the technique works well to evoke responses to modulation frequencies between 70 and 110Hz and that four more stimuli can be simultaneously presented without significant reduction of amplitude in carrier frequency separated by one octave, in intensities below 60dB.
Rance et al. (1998) studied 108 children who did not present short latency evoked potentials for 100dB HL clicks through steady-state evoked potentials in frequencies from 250 to 4000Hz and compared these results to behavioral thresholds; they concluded that amplitude-modulated tones used in SSEP allow search for specific frequencies in loud intensity and that there is a close correlation between them and pure tone thresholds.
Valdes et al. (1997) demonstrated that four different statistical methods (CSM, CT2, T2H and F test) could be similarly used to objectively detect the frequency peaks that characterize steady-state evoked potential responses. According to them, the efficient method to standardize the signal-noise ratio in the frequency domain, which used the representation of polar coordinates of complex values, resulting from Fast Fourier Transformation (FFT), is based on differential behavior of signal and noise during promediation and uses two different statistical calculations: attenuation and synchrony, based on amplitude and phase values, respectively (Figure 2).
According to Jerger (1998), auditory assessment by SSEP bridges a gap between the positive proprieties of transient brainstem evoked potentials and the excessive dependency of CNS conditions for late frequency-specific evoked potential.
SSEP provides essential information to guide therapy and rehabilitation management to be effective in neonate and infants hearing loss or in patients that do not cooperate in conventional audiometry (Rance; Dowell; Rickards, 1998).
The purpose of the present study was to analyze the responses obtained by steady-state evoked potentials in normal subjects and to compare them to the results reported in the literature, trying to show the importance of including the technique in the routine of objective audiometric assessment.
MATERIAL AND METHODThis study was conducted at the Service of Audiology, Human Communication Disorder Clinic, University of North Parana, UNOPAR, using equipment Audix - version 2.0, installed at Audiologus - Hearing and Voice clinic, in Londrina - PR, between October and December 2000.
We analyzed SSEP obtained for 25 adult subjects, 21 female and 4 male subjects, aged from 21 to 40 years, with no hearing complaints and submitted to air pure tone audiometry in frequencies 250, 500, 1000, 2000, 3000, 4000, 6000 and 8000Hz, with thresholds equal or below 15dB HL.
Integrity of brainstem hearing pathways was checked by search for BERA absolute and relative latency at 75dB HL intensity (ASHA, 1988).
We analyzed minimal levels of response of SSEP stimulated by complex acoustic signal, formed by frequencies of 500, 1000, 2000 and 4000Hz, modulated in amplitude, with modulation frequency of 104, 107, 111 and 115Hz, respectively, with 20 and 40dB HL intensity.
Electrodes were placed as follows: active electrode on the vertex (Cz); reference electrode on the ipsilateral mastoid, and grounding electrode on the contralateral mastoid, and recordings were made with subjects seated on a comfortable reclining chair, fully relaxed or slightly asleep, without the use of sedation.
The stimulus was presented monaurally for approximately 9 seconds for each multi-frequency stimulus. We conducted a minimum of 30 and a maximum of 50 promediation. Thus, it took approximately 6 minutes to record each studied frequency (20, 25, 30, 35 and 40dBHL) by ascending order up to the point in each the responses were recorded for each frequency. The average time spent to assess SSEP for each subject was about 45 minutes.
The presence of SSEP responses in the frequency domain was calculated based on the analysis of amplitude and spectral component phase generated by multi-frequency stimuli (500, 1000, 2000 and 4000Hz) modulated in amplitude, and the peaks of frequency corresponding to modulation frequencies that were statistically greater than noise levels, using the T2H statistical method, were considered valid.
Recording of evoked responses was interrupted or we excluded the data whenever the noisefloor activity level exceeded 25mV or when impedance could not be maintained below 5KW.
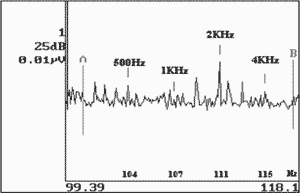
Figure 1. SSEP responses characterized by spectral components represented by peaks that correspond to modulation frequencies.
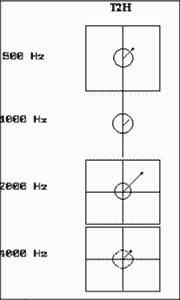
Figure 2. Objective analysis of SSEP responses using statistical method (T2H) to check the signal/noise ratio in the frequency domain, indicating the presence of SSEP in frequencies 500Hz, 2K and 4KHz and absence of response in frequency 1KHz.
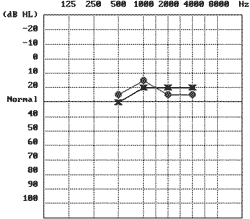
Figure 3. "Electrophysiological audiogram" represented by SSEP responses.
ResultsAll studied subjects presented responses to SSEP in both ears for modulated amplitude tones, within 20 to 40dB HL intensity, in at least 6 of the 8 studied frequencies (4 in each ear). The evoked potentials in the frequency domain are characterized by spectral peaks, statistically higher than noise level, found in frequencies identical to the modulation, that is, 104Hz for the carrier frequency 500, 107Hz for 1kHz, 111Hz for 2kHz and 115 for carrier frequency 4kHz.
For a total of 200 analyzed frequencies in 25 studied subjects, we detected 196 minimal levels of response in the intensity range (20 to 40dB HL). Thus, the percentage of valid responses found in 97% of the total expected responses were distributed as follows: 96% in 500Hz; 96% in 1kHz, 100% in 2kHz, and 96% in 4kHz.
A significant concentration (85%) of the potentials was obtained with stimuli between 20 and 30dB HL, to wit: 80% in 500Hz, 84% in 1kHz, 90% in 2kHz, and 86% in 4kHz (Graph II).
There was a reduced percentage of responses (13%) that were identified in intensities between 35 and 40dB HL. Out of this total, 10% were at 35dB HL, as: 14% in 500Hz, 10% in 1kHz, 6% in 2kHz and 10% in 4kHz, and only four responses to SSEP were evoked in 40dBHL, corresponding to 3% of the studied frequencies. For the 4kHz frequency, no response was registered at this intensity (Table 1).
There were still 4 subjects, all female, who did not present response in one or two of the frequencies, totaling 2% of the recordings. Of these, 2 did not present response in only one frequency, one in frequency of 1kHz on the right ear and the other in 4kHz on the left; other 2 subjects did not present response in 2 of the frequencies in one of the ears: one in frequency of 0.5 and 1kHz and the other in 0.5 and 4kHz, both on the left. In such cases, data collection was interrupted before the responses were recorded, since the present study was limited to a maximum of 50 promediation and maximum intensity of 40dB HL.
Minimum levels of responses of SSEP obtained in this sample presented the following mean intensity values: 26.60dB HL for frequency 500Hz; 25.63dB HL for 1KHz; 26.80dB HL for 2KHz and 25.20dB HL for 4KHz on the right ear, and 27.17dB HL for 500Hz; 24.79dB HL for 1KHz; 25.00dB HL for 2KHz and 24.57dB HL for 4KHz on the left ear.
Considering all the frequencies studied on both ears, the average of intensities necessary to evoke SSEP responses was 25.72dB HL, within a reduced range (24.57 and 27.17dB HL). It is important to emphasize that these values should not be considered as the average of SSEP thresholds, since there were no intensities below 20dB HL, so mean estimated values for SSEP thresholds should correspond to intensities below those above-reported.
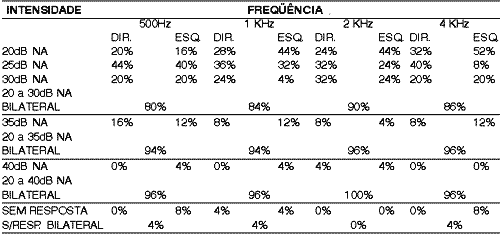
Table I. Percentage distribution of responses by frequency and ear in each stimulus intensity and accumulated percentage of bilateral responses for different intensity ranges.
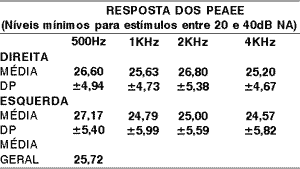
Table II. Mean values of minimum responses to SSEP obtained for each frequency.
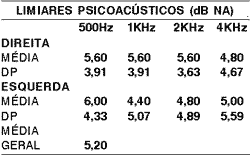
Table III. Mean values of psychoacoustic thresholds between 500 and 4000Hz, from each frequency and total of assessed frequencies.
DiscussionThe improvement of procedures used to objectively assess hearing is especially important in cases of small or newborn children who present signs of abnormalities in the universal neonatal hearing screening recommended until the third month of life. Hearing loss is a serious health problem in childhood owing to the damage it causes to language and speech development and, so as to have the appropriate therapeutic and rehabilitative approach to minimize the problems triggered by sensorial deprivation, early detection and characterization of hearing deficit is required.
Among the most frequently used procedures in objective audiological assessment, we can highlight immittanciometry, evoked otoacoustic emissions and BERA.
These tests, used together, provide important data about type and severity of hearing loss when behavioral assessment is not conclusive; however, even in some cases the information is not enough to correctly characterize hearing.
Spectral components of steady-state evoked potentials by amplitude-modulated tones, found in all subjects of the current sample, in modulated frequencies of 104 to 115Hz, long duration stimuli, which are more frequency-specific than clicks, allow the estimation of psychoacoustic thresholds, within reasonable approximation, in the 4 studied frequencies (0.5, 1K, 2K and 4kHz) considered the most important ones for speech sound reception, and proved to be easy to be identified, owing to objectively of the response analysis.
In the present study we did not emphasize the statistical comparisons between pure tone thresholds and minimal response levels of SSEP, since evoked responses by stimuli below 20dB HL were not studied to avoid prolonging assessment time. Even so, the results found (Tables II and III) which are probably above the mean estimated values for thresholds of SSEP, were compatible to those found by Lins, 1996; Valdés, 1997; Jerger, 1998 and Rance, 1998, among others, since the mean responses were found in 25.72dB HL and the pure tone mean threshold of the sample was 5.20dB HL, that is, the difference between them was approximately 20dB HL.
We did not conduct statistical analysis by gender or age since according to the studied literature, these variables were not relevant for adult groups.
The increased activity of EEG which hinders the collection of electrophysiological responses of short amplitude owing to increase in noise, normally caused by an unsatisfactory relaxation state, can be considered the main causal factor for absence of responses detected in 4 subjects or 2% of the recordings. The use of sedation, routinely administered to small children or patients that do not collaborate, are recommended to solve this problem.
In view of the results found in the present study, applicability and usefulness of SSEP in objective audiometry assessment could be confirmed, especially concerning the audiometric profile, enabling configuration of the electrophysiological audiometry (Figure 3). However, it was clearly realized that SSEP study does not provide information about the site of the hearing damage, that is, the present method should not be used isolated, since it is a technique that supplements but does not replace other assessment approaches previously mentioned (immittanciometry, otoacoustic emissions and BERA), which are valuable tools to the same end.
ConclusionThe analysis of steady-state evoked potential responses obtained from a sample of normally-hearing subjects showed results compatible with those of studies conducted in other countries, and appoints to the possibility of estimating objectively the auditory thresholds in frequencies 500, 1000, 2000 and 4000Hz, with average approximation of 20dB of psychoacoustic thresholds, within a timeframe compatible with the clinical practice (from 30 to 60 minutes). Based on the literature analysis and the data collected in the present study, we concluded that the main advantages of the method are the use of clear statistical methods that allow the determination of presence of response, excluding the subjectivity of tracing analysis, and the possibility of assessing various frequencies simultaneously. Thus, it was demonstrated that SSEP recordings are very useful to objectively assessing hearing.
Graph I
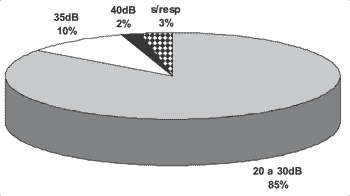
Graph II
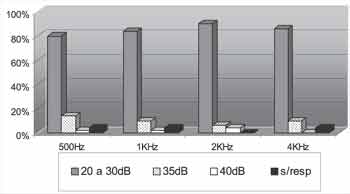
REFERENCES1. Aoyagi M, Kiren T, Furuse H, Fuse T, Susuki Y, Yokata M, Koike Y. Pure tone threshold prediction by 80-Hz amplitude-modulation following response. Acta Oto-laryngologica 1994;511 Supplement (Stockholm):7-14.
2. Aoyagi M, Kiren T, Kim Y, Suzuki Y, Fuse T, Koike Y. Optimal modulation frequency for amplitude-modulation following response in young children during sleep. Hearing Research 1993;65:253-261.
3. Conijin EAJG, Brocaar MP, Van Zanten GA. Frequency-specific aspects of the auditory brainstem response threshold elicited by 1000 Hz filtered clicks in subjects with sloping cochlear hearing losses. Audiology 1993;32: 1-11.
4. Conijin EAJG, Brocaar MP, Van Zanten GA, Van Der Drift JFC. Comparison between the frequency specificity of auditory brainstem response thresholds to clicks with and without high-pass masking noise. Audiology 1992;31:284-92.
5. Ferraro JA, Durrant DJ. Auditory Evoked Potentials: Overview and Basic Principles. In: Katz J. Handbook of Clinical Audiology. 4th ed. Baltimore: Willians & Wilkins; 1994. cap. 22 p.317-338.
6. Gorga MP, Kaminski JR, Beauchaine KA, Jesteadt W. Auditory brainstem responses to tone burst in normally hearing subjects. J Speech Hear 1988;31:87-97.
7. Hood LJ. Clinical Applications of the Auditory Brainstem Response - Introduction and Overview - Singular Publishing. San Diego; London 1998. Cap.1. p.3-9.
8. Jerger J. The auditory steady-state response. J Am Acad Audiology 1998;9(5): 314.
9. John MS, Lins OG, Boucher BL, Picton TW. Multiple auditory steady-state responses: stimulus and recording parameters. Am Acad Audiology 1998;9(5):315-31.
10. Lins OG, Picton PP, Picton TW, Champagne SC, Durieux-Smith A. Auditory steady-state responses to tones amplitude-modulated at 80 to 110Hz. Journal of the Acoustic Society of America 1995;97:3051-3063.
11. Lins OG, Picton TW, Boucher BL, Durieux-Smith A, Champagne SC, Moran LM, Perez-Abalo MC, Martin V, Savio G. Frequency-specific audiometry using steady-state responses. Ear and Hearing 1996;17:81-96.
12. Rance G, Dowell RC, Rickards F.W, Beer DE, Clark GM. Steady-state evoked potential and behavioral hearing thresholds in a group of children with absent click-evoked auditory brain stem response. Ear Hear 1998;19(1):48-61.
13. Richards FW, Tan LE, Cohen LT, Wilson OJ, Drew JH, Clark GM. Auditory steady-state evoked potentials in newborns. British Journal of Audiology 1994;28:237-337.
14. Stapells DR, Gravel SG, Martin BA. Thresholds for auditory brainstem responses to tones in notched noise from infants and young children with normal hearing and sensorineural hearing loss. Ear and Hearing 1995;16:22-34.
15. Stapells DR, Picton TW, Durieux-Smith A, Edwards CG, Moran LM. Thresholds for short-latency auditory evoked potentials to tones in notched noise in normal-hearing and hearing impaired subjects. Audiology 1990;29:262-274.
16. Van Der Drift JFC, Brocaar MP, Van Zanten GA. The relation between the pure-tone audiogram and the click auditory brainstem response threshold in cochlear hearing loss. Audiology 1987;26:1-10.
17. Valdés JL, Perez-Abalo MC, Martín V et al. Comparison of statistical indicators for the automatic detection of 80 Hz auditory steady state response. Ear Hear 1997;15:45-59.
STUDIED LITERATURE1. Champlin CA. Methods for detecting auditory steady-state potentials recorded from humans. Hearing Research 1992;6:144-150.
2. Dobie RA, Wilson MJ. Objective response detection in the frequency domain. Electroencephalography and Clinical Neurophysiology 1993;88:516-524.
3. Durieux-Smith A, Piction TW, Bernard P, MacMurray B, Goodman JT. Prognostic validity of brainstem electric-response audiometry (BERA) in infants of a neonatal intensive care unit (nicu). Audiology 1991;30:249-265.
4. Galambos R, Wilson M. Newborn hearing thresholds, measured by both insert and earphone methods. Journal of the American Academy of Audiology 1994;5:141-145.
5. Hyde ML, Matsumoto N, Alberti PW. The normative basis for click and frequency-specific BERA in high-risk infants. Acta Otolaryngologica 1987;103:602-611;361-371.
6. John MS et al. Human auditory steady-state responses to amplitude-modulated tones: phase and latency measurements. Hear Research http://www.sborl.com.br/html/body. boletins.htm, 2000.
7. John MS, Picton TW. A Windows program for recording multiple auditory steady-state responses. Comput Methods Programs Biomed 2000;61:2125-50.
8. Kraus N, Mc Gee T. Auditory Event-related Potentials. In: Katz J. Handbook of Clinical Audiology. 4. ed. Baltimore: Willians & Wilkins; 1994. cap. 27 p. 406-426.
9. Lins OG, Picton TW. Auditory steady-state responses to multiple simultaneous stimuli. Electroencephalography and Clinical Neurophysiology 1995;96:420-432.
10. Martin BA, Boothroyd A. Cortical auditory evoked potentials in response to changes of spectrum and amplitude. Journal of the Acoustic Society of America 2000;107(4):2155-61.
11. Picton TW, Durieux-Smith A, Moran LM. Recording auditory brainstem responses from infants. International Journal of Pediatric Otorhinolaryngology 1994;28:93-110.
12. Rance G, Rickards FW, Cohen LT, De Vidi S & Clark GM. The automated prediction of hearing thresholds in sleeping subjects using auditory steady-state evoked potentials. Ear and Hearing 1995;16:499-507.
13. Starr A, Don M. Brain Potentials Evoked by Acoustic Stimuli. In: TW Picton (Ed.) Human event-related potentials. Handbook of electroencephalograpy and clinical neurophysiology. Vol. 3, Amsterdam: Elsevier; 1988. p.97-157.
14. Stein LK, Jabaley T, Spitz R, Stoakley D, McGee T. The hearing-impaired infant; patterns of identification and habilitation revised. Ear and Hearing 1990;11:201-205.
15. Valdés M. Pesquisaje de defectos auditivos en lactentes mediante potenciales evocados auditivos de tallo cerebral. Tesis para optar por el grado de Doctor en Ciencias Médicas. CINC, La Habana, Cuba, 1985.
16. Victor JD, Mast J. A new statistic for steady state evoked potentials. Electroencephalography and Clinical Neurophysiology 1991;78:378-388.
17. Weber BA. Auditory Brainstem Response: Threshold Estimation and Auditory Screening. In: Katz J. Handbook of Clinical Audiology. 4th ed. Baltimore: Willians & Wilkins; 1994. cap. 25, p.375-386.
18. Wernwe LA, Folsom RC, Mancl LR. The relationship between auditory brainstem response and behavioral thresholds in normal hearing infants and adults. Hearing Research 1993;68:131-141.
19. White KR, Behrens TR. The Rhode Island hearing assessment project: Implications for universal newborn hearing screening. Seminars in Hearing 1993;14:1-119.
[1] Otorhinolaryngologist, Professor of Speech and Language Pathology and Audiology, UNOPAR.
[2] Speech and Language Pathologist and Audiologist, Professor of Speech and Language Pathology and Audiology, UNOPAR.
[3] Speech and Language Pathologist and Audiologist, Master Degree, Professor of Speech and Language Pathology and Audiology, UNOPAR.
Affiliation: University of North of Parana (UNOPAR) - Avenida Paris, 675 Jardim Piza - Londrina PR 86041-100
Address correspondence to: Luciana Lozza de Moraes Marchiori - R. Paranaguá 199 apto 901
Londrina - PR 86020-030 - E-mail: fono@unopar.br