INTRODUCTIONMultichannel cochlear implant (CI) is a highly beneficial and effective resource for the rehabilitation of subjects with sensorineural hearing loss of severe and profound levels. The CI comprises external and internal components. The external components are microphone, speech processor and transmitting antenna. Among the internal components we can include: receptor-stimulator, which includes an internal antenna, surgically placed close to the skull base bones, behind the ear under the skin, and an electrode bundle, which is positioned in the cochlea. The CI partially replaces the cochlear functions, transforming sound energy into electrical signals. The survival of sufficient neural structures in the cochlear nerve allows that the electrical stimulation be sent to the cerebral cortex.
An important factor for CI is that the necessary amount of current to evoke a hearing sensation is different in each subject and each stimulation channel. Therefore, the electrical stimulation parameters, in the speech processor, should be individually adjusted to suit the needs of users. It is conducted by a process named "mapping". The more accurate the mapping, the higher the potential for the patient to achieve speech perception in a open set 1, 2. Mapping is conducted by speech therapists, using a programming system that comprises a PC connected with a specific interface, which in turn, is connected to the speech processor through a cable. By using the programming software, the speech therapist can access the interface and send signals, at precisely determined levels, to the speech processor, and then, to the patient 3.
The common procedure in programming cochlear implants is the determination of the dynamic area for electrical stimulation. The dynamic area is the region comprised between the amount of current that induces hearing sensation, that is, the threshold of electrical stimulation (level T) and the level of sensation of maximum intensity that the patient is going to accept for electrical stimulation (C level). It is done so that CI can be programmed within a loudness extension that allows all speech sounds and other sounds to be audible but not uncomfortable 4.
The dynamic area is determined by psychophysical measures; the therapist depends on a number of factors, such as age, mental status, type of hearing loss and other developmental aspects of the patient 2. In small children or subjects with other associated impairments, the definition of the dynamic range is part of a long and complicated process, especially in initial periods of CI use. The limited hearing experience that these subjects had before the cochlear implant surgery, as well as cognitive and linguistic maturity to conduct the necessary procedures results in inconsistent responses in some occasions. Even though these conditioning and behavioral observation techniques can be used to reach levels T and C, unfortunately, for a part of the population, these values are not collected in a reliable fashion. As a result, these levels are many times arbitrarily adjusted.
Recent improvement in technology of cochlear implant and the results of speech recognition have led to an expansion of the criteria or the selection of cochlear implant surgery candidates, comprising audiological criteria as well as age at surgery. Recently, children as young as 12 months old have been implanted. Studies show that these children with congenital hearing loss that were implanted early have better results of speech recognition 5, 6.
The younger surgical age gave rise to the need for objective measures in order to stimulate the psychophysical levels of electrical stimulation appropriate to program the speech processor. Studies are conducted with evoked auditory brainstem responses (ABR) recorded in implanted subjects in order to correlate the thresholds of the potentials with levels T and C 7, 8. ABR recording requires the use of surface electrodes and can be easily contaminated by muscle activity artifacts or external electrical activity. Owing to that, it is difficult to have such recordings in small children that have not been sedated or are sleeping soundly or in environments not prepared to isolate environmental interference.
Stapedial reflex has been measured in response to a electrical stimulus to the cochlea by directly observing the contraction of the stapedial muscle in the surgical act or by the use of measures of acoustic impedance standards in the ear contralateral to the implanted side. The stapedial reflex thresholds electrically evoked can be used to estimate the C level, but such measures are widely variable both intra and inter-subjects. In addition, these reflexes are not recorded in approximately 40% of the population 9, 10.
A more direct way of measuring the cochlear nerve function is using Electrically Evoked Compound Action Potential (ECAP). ECAP reflects the synchronized firing of cochlear nerve fibers and it is very much similar to the forms of wave I found in the ABR, occurring in latencies greater than 0.5ms 11. In humans, originally these measures used to be made only intra-operatively or by using cochlear implants that used percutaneous stimulation.
Neural Response Telemetry (NRT) is a new technique that allows the direct measure of ECAP intra and post-operatively in implanted patients. Currently, only the multichannel cochlear implant Nucleus 24 (Cochlear Co) provides this method, but it is estimated that in the future, other manufacturers of cochlear implants will add this capability to their implants 12. The measures made by NRT do not require additional equipment in addition to the one used in the mapping process, does not require sedation, few pro-measurement and can obtain it in approximately 80% of the studied subjects.
The NRT technique can be a useful tool in confirming integrity of internal devices, in objectively determining which electrodes can be included in a specific map, the best stimulation speed and the speech codification strategies, as well as the stimulation of levels C and T, which would be of extremely clinical importance 12.
The purpose of the present article was to describe the technique of neural response telemetry and some clinical applications and the survey in the area of cochlear implants.
LITERATURE REVIEWThe system of the Nucleus 24 has three telemetry systems: a system used to measure the impedance of individual electrodes in the implant, another system is used to monitor the compliance of current generators and a third system is the neural response telemetry (NRT) 13.
The measuring system of the NRT consists of the following segments: (1) computer with Windows system 3.11 or Windows 95, that is compatible with software WinDPS; (2) software NRT version 2.04 or superior; (3) programming interface; (4) speech processor and headset SPrint; (5) implant Nucleus 24. NRT software was developed by the department of engineering of Cochlear Corporation together with Abbas and Brown, University of Iowa, and was written by Dillier et al., in Zurich, Switzerland 12.
Figure 1 shows how the system works. A computer equipped with a programming interface is used to stimulate specific electrodes in the cochlea. A series of pulses of radiofrequency are transmitted from the interface of the speech processor SPrint to the internal receptor-stimulator used to evoke ECAP. The internal receptor-stimulator of IC Nucleus 24 is equipped with an amplifier and an analog-digital converter. These additional components allow that the voltage recorded with a pair of intra-cochlear electrodes be amplified, sampled and transmitted back to the external antenna and later to the programming interface. These voltages are analyzed and the resulting ECAP wave is shown on a screen and can be stored in the PC. No surface electrodes are needed to make the recording 13, 14.
The NRT system allows the stimulation and recording of any pair of electrodes, in monopolar and bipolar modes. Normally, the monopolar mode is used. The stimulation pulses are presented to a specific intracochlear electrode whose reference is the extracochlear electrode MP1, positioned right below the temporal muscle flap. Another intracochlear electrode is situated in the adjacent area and used as recording electrode, having as reference another extracochlear electrode (MP2), located in the receptor-stimulator. In general, the intracochlear electrode used to record ECAP is located approximately 1.5mm more apically (space between the two electrodes) in relation to the stimulation electrode. For example, if the selected stimulation electrode is the number 5, the recording electrode will be the number 7. The pair of active and reference electrodes used to stimulate should be different from the pair of electrodes used for the recording, in order to reduce artifact. Electrodes with high impedance values or electrodes that are not within the right compliance should not be used for the measurements 11, 14, 15.
ECAP is characterized by a single negative peak (N1) that occurs in one latency of approximately 0.2 to 0.4ms relative to the onset of stimulation. This negative peak is followed by a positive peak (P2). ECAP amplitude is measured between peaks N1 and P2 (Figure 2). Even though these amplitudes vary according to level of stimulation, position of electrodes and among the subjects, they can reach values of hundreds of µV.
The stimulus used to evoke ECAP is a biphasic current of 25µs/phase presented at stimulation ratio of 400Hz, but speeds of 80Hz or 250Hz are normally recommended 15. The system was developed to record a series of 16 samples after each pulse of stimulus and the maximum sampling ratio was 10kHz. The internal amplifier gain can be adjusted to 40, 60 or 80dB, being recommended 60dB as the standard. The amplifier output is sampled and transmitted to the external antenna as 16 pairs of pulses. The time between the pairs of pulses carries information of voltage and it is limited between 8 and 9 bits of resolution 13. Since ECAP is registered in the cochlea it is necessary to have little promeasurement, normally using from 100 to 200 scans. The response is not affected by the muscle artifact, which can be registered without sedation. The disadvantage of this procedure is that the artifact of the electrical stimulation, higher in magnitude than the neural response, is recorded exactly with it. Therefore, the separation between the neural response of the artifact and the stimulus becomes mandatory 16, 17.
The subtraction method 18, 19 uses the refractory proprieties of the cochlear nerve to reduce artifact in the NRT. The action potential in a nervous fiber is followed by a brief period of reduction of excitability, or refractory period, which can be divided into two stages: absolute and relative. The absolute refractory period occurs immediately after the action potential. During this period, it is impossible to excite again the cell, no matter how large the magnitude of the applied stimuli 20, 21.
In general, the subtraction technique requires two pulses of biphasic current, a probing stimuli and a masking stimulus, presented at sufficient speed and level to make the nerve go into the refractory period, and therefore, it is possible to subtract the neural response to the artifact generated by the stimulus. The paradigm of the subtraction involves the following intervals of stimulation (Figure 3).
a) only the stimulus (probing) is presented resulting in a record that consists of neural response and artifact generated by the device;
b) presentation of the masking stimulus followed by a short time interval (named inter-pulse interval - IPI), of the same probing stimulus used before. An IPI of 0.3 to 0.5ms is appropriate to ensure the paradigm of subtraction 22. It is supposed that if the interval between the masking and the probing stimuli is sufficiently short, the neurons that normally fire the probing stimuli are in the absolute refractory period because they had already fired in view of the masking stimulus. It produces a recording that has neural response and artifact coming from the presentation of the masking stimulus, as well as only the artifact, originated from the presentation of the probing stimulus;
c) masking and probe stimuli are presented again, but the probing stimulus is adjusted at a minimum level, which effectively results in conditions of the presentation only of the masking stimulus. The record corresponds to artifact coming from the masking stimulus and the neural response.
If we subtract C minus B, we will have the value of the artifact, generated by the stimulation of the probing. If we subtract the artifact from condition A, we will have the condition generated by the probing stimulus. Another artifact is produced whenever the amplified used in the measurement is on. This artifact is recorded in four intervals:
d) presentation of the masking and probing stimuli in minimum amplitudes, and therefore, it does not contribute to generating artifacts. This artifact of the amplifier will be subtracted from the other records.
Summing up, the neural response will be equal to: A - (B - (C-D)).
It is not possible to know in advance what is the level of the masking stimuli that will be enough in each measuring. However, the presentation of the masking stimulus with an amplitude equal or greater than the probing stimulus increases the likelihood of having efficient masking 13.
The presentation level of masking and probing stimuli should be sufficiently high to allow a measurable neural response, but it is also imperative that the stimulation does not exceed the tolerance level (maximum acceptable level - NMA). NMA represents the upper limit of stimulation to make the recording and should not be exceeded 15.
There are a number of parameters that have to be selected when using telemetry of neural responses for the record of ECAP. The manipulation of these parameters affects the responses obtained and, therefore, it is determinant that they be appropriately adjusted, according to the objective that is intended. The readers can access the original articles 12, 13, 15, 17, 23 to check the protocols proposed for the optimization and record of ECAP. The parameters that are being used in a given measurement can be visualized during the test (Figure 4, panel A).
Amplitude increasing curves:
The latency of electrically evoked responses is not affected by the level of stimulus presentation. Thus, wave amplitude is normally used to quantify the ECAP. By means of this resource, the software marks each curve, in which there are minimum and maximum amplitudes. The marking is indicated by cursors and can be visualized in a graph of amplitudes by time (Figure 4, Panel D).
The values of amplitude of response for each one of the different levels of probing stimuli are recorded in a graph. A line of linear regression is designed using the algorithm presented in the NRT software (Figure 4, panel C). The intersection point between this line and the axis shows the extrapolated threshold value, denominated pNRT 12. ECAP threshold can also be visually determined.
Studies conducted with ECAP in implanted patients, in the pre and post-surgical period, demonstrated that there are variations both of thresholds and growth curves of amplitude between the subjects and the electrodes in the same subjects 14, 24. It suggests that such measures can be indicative of the threshold and spatial distribution of the surviving neural population that is being affected for each electrode.
Investigations conducted showed that in most subjects, the thresholds of ECAP are obtained in values closed to level C obtained in the mapping 12, 17, 28, 23. However, there is variability of these results between subjects and electrodes in the same subject. Correlation of the thresholds of ECAP with levels T and C were found in the case of children 17. In general, the studies concluded that thresholds of ECAP obtained in the NRT can serve as the basis to adjust the levels of comfort in the maps, being suggested the use of NRT together with behavioral measures obtained in only one electrode, in order to facilitate the programming of the speech processor 23.
Recovery curves
The capacity of cochlear nerve fibers to accurately transmit temporal information can affect the performance of the implant use. A way of assessing the temporal processing capacity of a user of cochlear implant is to measure the refractory properties of the cochlear nerve. These properties refer to the effect of a stimulus on the response to a subsequent stimulus and can be extremely important in determining the capacity of neurons to reproduce complex temporal patterns of stimuli necessary to codify speech 24.
Refractory properties can be measured using NRT using the subtraction technique. In this case, the probing and masking stimuli can be maintained fixed at a supraliminary level and inter-pulse interval (IPI) is variable. The IPI variation allows the analysis of the effects of the variations of stimulus temporal properties on ECAP, defining the so-called recovery curves. As IPI increases, there is more time after the masking sound for the neurons to get out of the refractory period and respond to the probing stimulus, in the intervals used in the subtraction paradigm. The consequence is that amplitude of subtracted responses will reduce as there is increase in inter-pulse interval.
Initial studies demonstrated heterogeneity in recovery curves, both among subjects and electrodes in the same subject 14, 18. Other studies are necessary to relate these data with the performance of implanted subjects.
DISCUSSIONCochlear implants have different numbers and positioning of intra-cochlear electrodes and allow the selection of parameters to be adjusted in each electrode in a specific way. With the expansion of the selection criteria, the use of objective measures to support programming of speech processing became recommendable.
In addition, the data correlated with permeability of the cochlear nerve to electrical stimulation and the way in which some parameters of electrical stimulation interact with the surviving neural structure is still a challenge for professionals that work in a program of cochlear implant. The determination of stimulating neural elements that already exist in different pathological processes of the cochlea can also be of great use since it is supposed that survival of ganglionar cells of other neural elements can be one of the causes of the variability in relation to performance of speech recognition found in implanted subjects.
Neural response telemetry can be an advantageous method for the study of these issues since 13, 14, 16, 18, 24:
ECAP is the most direct measurement of cochlear nerve function in humans and, as such, can be effective to analyze the abnormalities of the auditory system resulting from peripheral hearing loss, as well as the stimulation standards of the cochlear nerve and how they vary in subjects and depend on the stimulation site along the cochlea;
the recording made by intracochlear electrodes allow capturing of responses with higher amplitudes than when recorded by surface electrodes, which reduces the number of screenings necessary to collect the data, making it a quicker and more efficient procedure. In addition, minor muscle activity does not contaminate the recordings, dispensing sedation, which makes it easy to be used in children;
ECAP has relatively short latency time, which allows the verification of abnormalities obtained in the response to high-speed stimulation that is used in regular speech processors.
Conversely, this measure presents some restrictions. For example, ECAP does not reflect the alterations that occur at the level of the central nervous system resultant from peripheral hearing loss or subsequent electrical stimulation To assess them and other issues concerning the upper processing functions, it is necessary to use other electrophysiological measures, such as late potentials.
CLOSING REMARKSNeural response telemetry is a new technique that allows recording of the action potential comprising peri- and post-operative electrically evoked situations in users of Nucleus 24 cochlear implant. Many research centers are studying the use of these procedures to stimulate thresholds and comfort levels for electrical stimulation using speech processor programming. Other studies are needed to understand how these measures can be used to support the study of properties of surviving structures of the cochlear nerve.
FIGURES
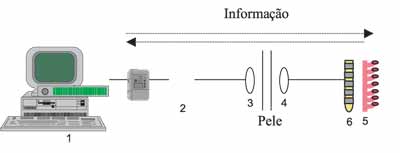
1: Graphic representation on how ECAP can be measured using neural response telemetry in Nucleus 24 cochlear implant: (1) computer and programming interface; (2) speech processor; (3) external antenna; (4) receptor-stimulator; (5) electrode bundle, and (6) cochlear nerve.
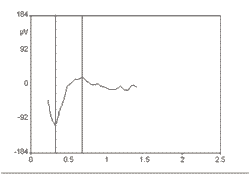
Figure 2: Representation of ECAP measured by the neural response telemetry technique.
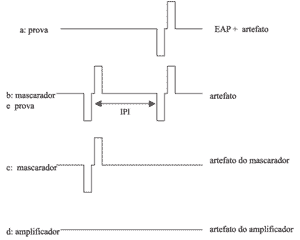
Figure 3: Intervals used in the subtraction paradigm.
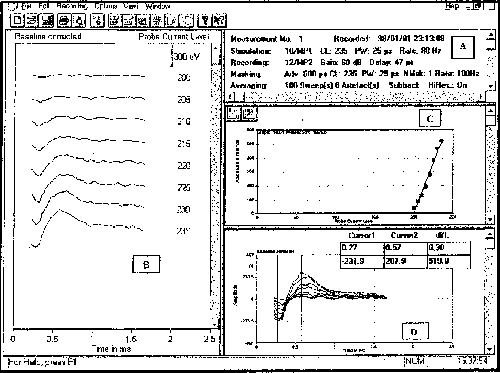
Figure 4: Panel A: Simulation parameters and records of use to measure ECAP in electrode 10 and level of stimulation equal to 235. Panel B: Different amplitudes of ECAP obtained with variation of probing stimulus. Panel C: Graph of amplitudes of ECAP depending on levels of probing stimulus and regression curve provided by NRT software. Panel D: Response of ECAP to stimulation level 235, using cursors positioned by software NRT, v. 2.04.
REFERENCES1. Rance G, Dowell RC. Speech processor programming. In: Clark GM, Cowan RSC, Dowell RC. (eds.) Cochlear implantation for infants and children-advances. San Diego: Singular Publishing; 1997. p.147-70.
2. Shapiro W. Device programming. In: Waltzman SB, Cohen NL. (eds.). Cochlear implants. New York: Thieme; 2000. p.185-98.
3. Bevilacqua MC, Moret ALM. Reabilitação e implante coclear. In: Lopes Filho O. (ed.). Tratado de fonoaudiologia. São Paulo: Roca; 1997. p. 401-14.
4. Roberts S. Speech-processor fitting for cochlear implants. In: Cooper H (ed.) Cochlear implants: a practical guide. London: Whurr Publishers; 1991. p.201-18.
5. Bevilacqua MC. Implante coclear multicanal: uma alternativa na habilitação de crianças surdas. Bauru; 1998. 115p. (Tese de Livre Docência - Faculdade de Odontologia de Bauru Universidade de São Paulo).
6. Tyler RS, Fryauf-Bertschy H, Kelsay DM, Gantz BJ, Wooodworth GP, Parkinson A. Speech perception by prelingually deaf children using cochlear implants. Otolaryngol Head Neck Surg 1997; 117:180-7.
7. Fria TJ, Shallop JK. Application of evoked potential audiometry to children with cochlear implants. In: Owens E, Kessler DK. Cochlear implants in young deaf children. Boston: College-Hill; 1989. p. 71-80.
8. Hodges AV, Ruth RA, Lambert PR, Balkany TJ. Electric auditory brain-stem responses in nucleus multichannel cochlear implant users. Arch Otolaryngol Head Neck Surg 1994; 120:1093-99.
9. Spivak LG, Chute PM. The relationship between electrical acoustic reflex thresholds and behavioral comfort levels in children and adult cochlear implant patients. Ear Hear 1994; 15:184-92.
10. Spivak LG, Chute PM, Popp AL, Parisier SC. Programming the cochlear implant based on electrical acoustic reflex thresholds: patient performance. Laryngoscope 1994; 104:1225-30.
11. Shallop JK. Objective measures and the audiological management of cochlear implant patients. In: Alford BR, Jerger J, Jenkins HA. Electrophysiologic evaluation in otolaryngology. Basel: Karger; 1997. p.84-111.
12. Shallop JK, Facer GW, Peterson A. Neural response telemetry with the Nucleus CI24M cochlear implant. Laryngoscope 1999; 109:1755-9.
13. Abbas P, Brown C. Electrophysiology and device telemetry. In: Waltzman SB, Cohen NL. (eds.). Cochlear implants. New York: Thieme; 2000. p.117-51.
14. Brown CJ, Abbas PJ, Gantz BJ. Preliminary experience with neural response telemetry in the Nucleus CI24M cochlear implant. Am J Otol 1998; 19:320-7.
15. Lai W. An NRT cookbook: guidelines for making NRT measurements. Zurich: Cochlear AG; 1999. 32p.
16. Abbas PJ, Brown CJ, Shallop JK, Firzt JB, Hughes ML, Hong SH, Staller SJ. Summary of results using the Nucleus CI24M implant to record the electrically evoked compound action potential. Ear Hear 1999; 20: 45-59.
17. Hughes ML, Brown CJ, Abbas PJ, Gantz BJ. Using electrically evoked compound action potential thresholds to facilitate creating MAPs for children with the Nucleus CI24M. Adv Otorhinolaryngol 2000; 57:260-5.
18. Brown CJ, Abbas PJ. Electrically evoked whole-nerve action potentials: parametric data from the cat. J Acoust Soc Am 1990; 88:2205-10.
19. Sauvage CR De, Cazals Y, Erre JP, Aran JM. Acoustically derived auditory nerve action potential evoked by electrical stimulation: an estimation of the waveform of single unit contribution. J Acoust Soc Am 1983; 73:616-27.
20. Bear MF, Connors BW, Paradiso MA. Neuroscience: exploring the brain 2. Baltimore: Williams & Wilkins; 1996. p. 855.
21. Kandel ER, Schwartz JH, Jessel TM. Principles of neural science. 4.ed. New York: McGraw-Hill; 2000. 1414p.
22. Miller CA, Abbas PJ, Brown CJ. An improved method of reducing stimulus artifact in the electrically evoked whole-nerve potential. Ear Hear 2000; 21:280-90.
23. Hughes ML, Brown CJ, Abbas PJ, Wolaver AA, Gervais JP. Comparison of EAP thresholds with MAP levels in the nucleus 24 cochlear implant: data from children. Ear Hear 2000; 21:164-74.
24. Gantz BJ, Brown CJ, Abbas PJ. Intraoperative measures of electrically evoked auditory nerve compound action potential. Am J Otol 1994; 15:137-44.
1 Professor, Department of Speech Therapy and Audiology, FOB-USP; Speech Therapist, CPA-HRAC-USP Bauru -SP; Ph.D, studies in Neurosciences and Behavior under course, IP -USP, Sao Paulo.
2 Physician, Hospital de Reabilitação de Anomalias Craniofaciais (HRAC), USP, Full Professor, Department of Speech Therapy and Audiology, Dental School, Bauru (FOB) USP, Bauru-SP.
3 Full Professor, Head of the Department of Speech Therapy and Audiology, FOB-USP, Speech Therapist, HRAC-USP; Bauru-SP.
4 Professor, Department of Pathology and Laboratory of Functional Neurosurgery, Medical School, University of Sao Paulo, Sao Paulo.
Affiliation: Centro de Pesquisas Audiológicas do Hospital de Reabilitação das Anomalias Craniofaciais, University of Sao Paulo, Bauru-SP.
Financial support: Conselho Nacional para o Desenvolvimento Científico e Tecnológico (CNPq).
Address correspondence to: Deborah Ferrari - Al. Otavio Pinheiro Brizola 9-75 Vila Universitária Bauru SP 17043-900.
Tel (55 14)235 8332 - Fax (55 14)234 2280 - E-mail: dferrari@fob.usp.br